Leading Accelerator Technology
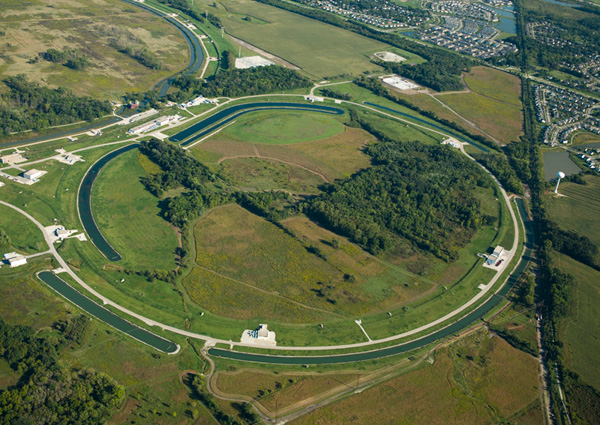
From blueprint to construction, Fermilab scientists and engineers develop particle accelerators to produce beams to take particle physics to the next level, collaborating with scientists and laboratories around the world to help build these complex machines. Researchers build accelerators to be efficient and robust along every step of the particle beam's path, from the time it's born to its termination on target.
The machines themselves must be efficient, cranking up beam to high energies while using as little energy as possible. They require strong magnets to form tight particle beams to get the most science out of them as they smash into targets or other beams. The targets must be robust and reliable, able to take the onslaught of high-power beams. And to see how it will play out before building the brick-and-mortar accelerator, computing experts simulate every last detail using advanced software and hardware, helping accelerator scientists build the right accelerator from the get-go.
Superconducting radio-frequency technology
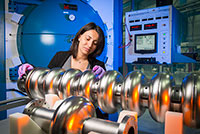
Fermilab tests and develops superconducting radio-frequency accelerating cavities, a key technology for next-generation accelerators and the future of particle physics.
SRF cavities enable accelerators to increase particle beam energy levels while minimizing the use of electrical power by all but eliminating electrical resistance. Future experiments looking into the origins of the universe and nature of matter, including the proposed International Linear Collider and Fermilab's new proton source, will require advanced SRF technology.
SRF technology is a highly efficient way to accelerate beams of particles. It starts with shiny, curvy, virtually perfect cells made of niobium, a superconducting metal, which are connected like a string of hollow pearls. The cells are cleaned and polished so that not a speck of dust or the slightest imperfections remain. Several strings, or cavities, are nestled in a vessel called a cryomodule, which bathes them in liquid helium and keeps them at the ultracold temperature that is key to their operation and efficiency.
Running through the string of pearls, or cells, is an electric field that oscillates between positive and negative millions or billions of times per second. Each cycle, or wave, swells to its peak and sinks to its valley within the space of a single cell; it is as if each cell rapidly switches between a positive and negative charge. The cycles are timed to kick charged particles as they speed from cell to cell. When the particles enter the cell they are pushed forward by the electric field; as the particles move to the next cell, the electric field switches so that the particles are again pushed forward once they enter that cell. This process continues until the particle has shot all the way through the accelerator.
To ensure that the accelerating field is as high as possible so that the particles get the high energy needed for the planned collisions, the cavities are welded, polished, high-pressure-rinsed and tested to the best performance.
Learn more about Fermilab's SRF program.
High-field magnets
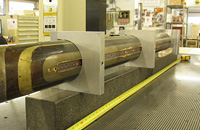
Magnets shape and steer the particle beam in a particle accelerator. They are used to make the beam bend around a curve and can also make the beam flat like a ribbon or round like a cord. The stronger the magnet — that is, the higher the magnetic field — the more focused the beam, the better the chances that particles will interact once they collide, whether they smash into a target or meet with another high-energy beam of particles coming at them.
Fermilab is one of the world's leading laboratories in accelerator magnet R&D. Scientists and engineers develop both superconducting and normal-conducting magnets to shape and steer beams at ever-higher energies. They are also working on new superconducting materials to develop even more powerful magnets for the next generation of particle accelerators.
A major component of Fermilab's magnet program is the development magnet technology for CERN's Large Hadron Collider in Geneva, Switzerland. The LHC Accelerator Research Program consists of four US laboratories that are working to develop accelerator components to increase the beam luminosity of the LHC.
Learn more about magnets at Fermilab.
Targetry
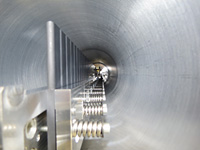
One way to flush out particles that are deeply hidden from view is to send an intense, powerful particle beam into a material, such as carbon or a metal, producing a gusher of new particles that scientists can then study. As the birthplace of particles in many particle physics experiments, the target is no less important than the beam that collides with it.
Fermilab experiments require hardy, robust targets that can withstand the power of the particle beams that pummel them. In the future, these targets will need to withstand even higher beam power and more punishment. Fermilab's scientists and engineers are hard at work developing targets and target materials that can resist the fatigue caused by the rapid and repeated punches and that retain their strength over long periods of time. Researchers are even investigating targets made out of liquid metals, which can withstand the very high-power beams envisioned for the future.
Fermilab is currently researching targets for the Deep Underground Neutrino Experiment and NOvA, as well as a proposed muon collider and neutrino factory.
Learn more about targetry at Fermilab.
Accelerator physics and modeling
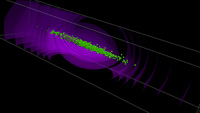
Accelerator theory and modeling expertise is of tremendous importance to accelerator design, research and development. Fermilab's talent in these areas helps accelerator experts make the most of their machines and plan for the future by giving them a better understanding of the physics of beams. Through theory and modeling, scientists also provide the tools to predict and simulate how a particle beam behaves when it is accelerated, steered and focused.
Beam physicists develop theoretical and analytical tools for the laboratory's current and next-generation accelerator facilities. They provide accelerator physics support for existing operational programs, train accelerator scientists and engineers, and carry out experimental programs for a broad range of accelerator R&D, which can be accessed by both Fermilab staff and the worldwide high-energy physics community.
Computer scientists develop software that lets experimenters manipulate the multiple variables involved in accelerating a beam of particles in a simulated setting. They run simulation programs on more than 100,000 cores, housed at Argonne National Laboratory, keeping Fermilab at the leading edge of the rapidly changing field of high-performance computing.
Experimenters currently apply beam dynamics and energy deposition simulations to the Main Injector for a future high-intensity proton accelerator, the debuncher for Mu2e, and studies of the Booster. They also contribute to simulations for the Large Hadron Collider in Geneva, Switzerland, and to the studies of Fermilab's future accelerator facilities.
Learn more about Fermilab's Accelerator Physics Center and beam simulations.
- Last modified
- 04/11/2016
- email Fermilab